As described in the prior Q&A gadolinium (Gd) exhibits powerful paramagnetism due to its electronic structure, possessing 7 unpaired electrons in its 4f shell. As these inner electrons are not directly involved with bonding, the paramagnetism of Gd is maintained even when formulated as a contrast agent attache to a larger molecule.
|
A further consequence of this electron structure is that the Gd+3 ion typically exhibits nine coordination sites for bonding and chemical interactions. In all MR contrast agents now commercially available, a ligand group occupies eight of these sites while the ninth is available for transient bonding by a solvent water molecule. This water molecule approaches very close (mean distance ~0.25 nm) to the metallic center, fitting into a crevice of the ligand. These direct and intimate magnetic interactions between water and the Gd+3 ion give rise to a process known as inner sphere relaxation.
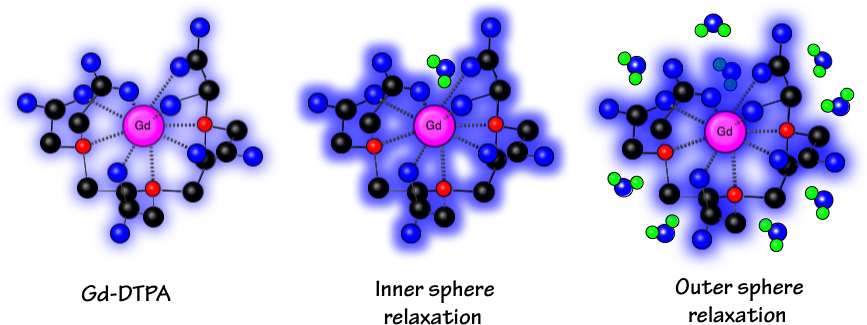
Ball and stick model of the contrast agent Gd-DTPA (Magnevist®). The Gd+3 ion is coordinated with 5 oxygen atoms (blue) and 3 nitrogen atoms (red). Structural carbon atoms are black. The crevice in the molecule leaves room for a single water molecule (blue and green) to interact directly with the Gd+3 ion (inner sphere relaxation). Beyond this a second shell of other water molecules experience outer sphere relaxation.
Advanced Discussion (show/hide)»
Although dipole-dipole interactions are primarily responsible for paramagnetic relaxation in the commonly used gadolinium chelates for clinical imaging, additional mechanisms may be important for other contrast agents, including non-gadolinium ones.
A second mechanism called the Fermi contact shift explains paramagnetic relaxation between an electron and nuclear spin. This involves the transfer of unpaired electron density to overlap with the s-orbitals at the center of the relaxing nucleus. It is a scalar process transmitted through bonds and very similar to nucleus-nucleus J-coupling. It is an important factor for the relaxation of certain transition element complexes such as low spin Fe (III) heme and Ni (II)-based contrast agents.
Finally, chemical exchange and magnetization transfer mechanisms can play important roles, especially explaining the properties of a new class of contrast materials called ParaCEST (Paramagnetic Chemical Exchange Saturation Transfer) agents. The paramagnetic center of these molecules is typically a non-gadolinium lanthanide-series element such as Eu (III) or Dy (III) complexed with ligands having easily exchangeable protons on −NH or −OH moieties. These ¹H protons chemically exchange first with coordinated water molecules in the outer sphere around the contrast agent, then subsequently transfer their magnetization to bulk water.
Aime S, Botta M, Fasano M, Terreno E. Lanthanide (III) chelates for NMR biomedical applications. Chem Soc Rev 1998; 27:19-29.
Belorizky E, Fries PH, Helm L, et al. Comparison of different methods for calculating the paramagnetic relaxation enhancement of nuclear spins as a function of the magnetic field. J Chem Phys 2008; 128:052315.
Caravan P, Ellison JJ, McMurry TJ, Lauffer RB. Gadolinium (III) chelates as contrast agents: structure, dynamics, and applications. Chem Rev 1999; 99:2293-2352.
De León‐Rodríguez LM, Martins AF, Pinho MC, et al. Basic MR relaxation mechanisms and contrast agent design. J Magn Reson Imaging 2015; 42:545-565.
Kruk D, Kowalewski J. General treatment of paramagnetic relaxation enhancement associated with translational diffusion. J Chem Phy 2009; 130:174104.
Yang C-T, Chuang K-H. Gd(III) chelates for MRI contrast agents: from high relaxivity to "smart", from blood pool to blood-brain barrier permeable. Med Chem Commun 2012; 3:552-565.
Can you explain a little more about the dipole-dipole interaction? I still don't quite understand.