Radiofrequency (RF) fields are part of the electromagnetic spectrum suitable for communication purposes, often cited to lie between about 3 kHz and 300 GHz. According to Maxwell's Laws, a changing magnetic field (B) is always accompanied by a changing electric field (E), and vice-versa. So the transmitted RF irradiation in MRI necessarily includes both B and E field components, which interact not only with nuclei, but with electrons, ions, atoms, and whole molecules simultaneously. Thus a wide range of potential effects of RF-field must be considered. These are normally classified as either non-thermal or thermal.
Non-Thermal Effects
Considerable controversy exists concerning whether non-ionizing irradiation in the RF spectrum causes genetic, cellular, or physiologic damage unrelated to tissue heating. Theoretical mechanisms of non-thermal injury include generation of reactive oxygen species ("oxidative stress") and alteration of calcium flux across cell membranes. Most research into this topic has focused on long-term or high intensity exposures, often at frequencies higher or lower than those used in MRI. Even the greatest skeptics would likely agree that non-thermal effects, even if they exist, are unlikely to be significant in the MRI setting. These issues are discussed in more detail with extensive references in another Q&A.
Thermal Effects
By contrast, thermal effects are universally recognized to produce a large number of potential biological changes, including impaired thermoregulation, alteration of chemical reaction rates, pain, and burns. Although most readers are probably aware that these effects exist, they may be surprised to learn the following:
- Only a tiny fraction (< 2%) of transmitted RF power is actually absorbed by nuclei.
- The absorbed nuclear energy transferred to the "lattice" via T1 relaxation makes a non-significant contribution to tissue heating.
- The coexistent electric field (E) -- not the magnetic field (B1) — is responsible for nearly all thermal effects in MRI.
More about the Electric Field (E)
The time-dependent E and B1 fields are related by the Faraday-Maxwell equation which can be written in differential form as: ∇ ✕ E = ∂B1/∂t. The left hand side of this equation contains a vector operator called "the curl" (∇ ✕), while the right hand side is a "derivative" (∂ /∂t), representing the time rate of change of B1. The electric (E) field can thus be visualized as "curling around" the magnetic (B1) field in concentric loops, whose properties depend on the instantaneous magnitude, direction, and time rate of change of B1. Both fields oscillate at the same frequency (ωo = 2πfo), so the direction of E is rapidly switching from clockwise to counterclockwise in each cycle.
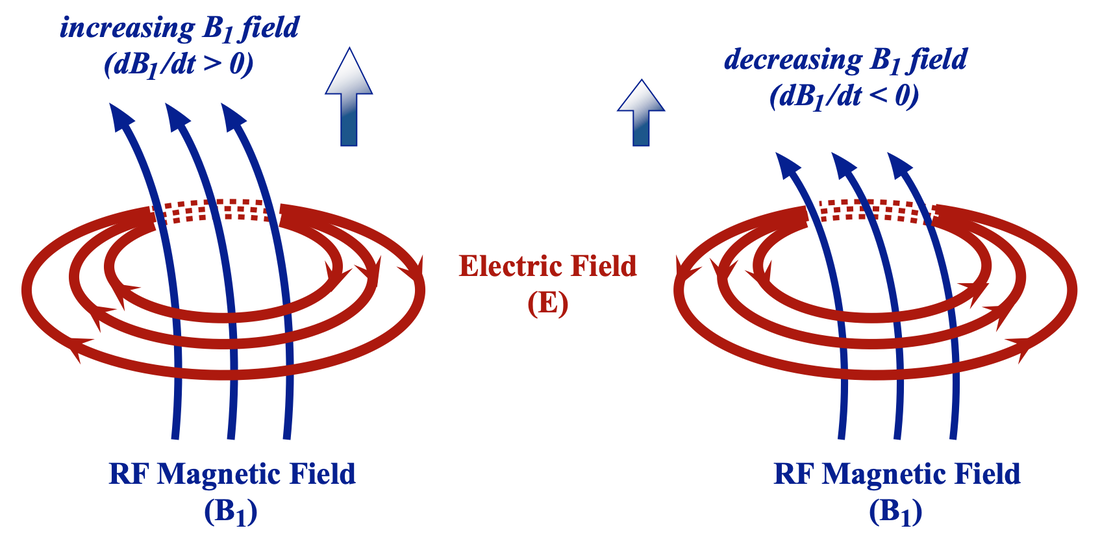
Relation of magnetic (B1) and electric (E) fields as defined by the Faraday-Maxwell equation. The lines of the E field "curl" around the B1 field. The direction of E is defined as the direction a hypothetical positive charge would move (or an electric current would flow) at a given point in space and time.
The E field deposits energy into tissues by two basic mechanisms: 1) resistive heating and 2) dielectric heating.
Resistive Heating
Pure water is a poor conductor, but biological tissues, which contain water plus dissolved electrolytes, conduct currents fairly well. The charge carriers in tissue are principally Na+, K+, Cl−, and HCO3− ions. Voltage gradients in the electric field accelerate these ions, imparting them with kinetic energy and creating an induced current. When these charge carriers collide inelastically with other ions, atoms, or molecules, their kinetic energy is transferred into random vibrational energy (thermal heat). This process is analogous to resistive loss of energy in a conventional electrical circuit governed by Ohm's Law. It is therefore alternatively referred to as Joule, Resistive, or Ohmic heating.
Dielectric Heating

Many molecules in the body are polar, especially water, based on its highly electronegative oxygen component. Polar molecules tend to align with an externally applied electric field. When the field changes, so do their orientations. In a rapidly changing RF E-field as exists in MRI, these rotating dipoles push and collide with other molecules, dispersing their kinetic energy as heat. This mechanism is effective over a large range of frequencies and is the same principle by which microwave ovens warm food.
The relative contributions of resistive and dielectric heating are difficult to separate out experimentally, as tissues are three dimensional and contain various components with different resistances and dielectric properties. It is generally believed that resistive heating is the more important mechanism at field strengths used in MRI, becoming progressively more dominant as radio frequencies increase.
Advanced Discussion (show/hide)»
No supplementary material yet. Check back soon!
References
Application Note. Basics of measuring the dielectric properties of materials. Keysight Technologies. Uploaded from www.keysight.com, Sept 2020
Chou CK, Bassen H, Osepchuk J, et al. Radio frequency electromagnetic exposure: tutorial review on experimental dosimetry. Bioelectromagnetics 1996; 17:195-208. [DOI link]
Foster KR, Glaser R. Thermal mechanisms of interaction of radio frequency energy with biological systems with relevance to exposure guidelines. Health Phys 2007; 92:609-620. [DOI link]
Gabriel C, Gabriel S, Corthout E. The dielectric properties of biological tissues: I. Literature survey. Phys Med Biol 1996; 41:2231-2249. [DOI Link]
Gabriel S, Lau RW, Gabriel C. The dielectric properties of biological tissues: II. Measurements in the frequency range 10 Hz to 20 GHz. Phys Med Biol 1996; 41:2251-2269. [DOI Link]
Gabriel S, Lau RW, Gabriel C. The dielectric properties of biological tissues: III. Parametric models for the dielectric spectrum of tissues. Phys Med Biol 1996; 41:2271-2293. [DOI Link]
Hoult DI. Sensitivity and power deposition in a high-field imaging experiment. J Magn Reson Imaging 2000; 12:46-67. [DOI link]
Miklavčič, D, Pavšelj N, Hart FX. Electric Properties of Tissues. In: Wiley Encyclopedia of Biomedical Engineering, M. Akay (Ed), 2006:1-12. [DOI link]
Payne D, Klingenböck A, Kuster N. IT’IS Database for thermal and electromagnetic parameters of biological tissues, Version 4.0, 15 May 2018. [DOI Link]
Schwan HP, Li K. Capacity and conductivity of body tissues at ultrahigh frequencies. Proceedings of the IRE 1953; 41:1735–1740. [DOI link]
Application Note. Basics of measuring the dielectric properties of materials. Keysight Technologies. Uploaded from www.keysight.com, Sept 2020
Chou CK, Bassen H, Osepchuk J, et al. Radio frequency electromagnetic exposure: tutorial review on experimental dosimetry. Bioelectromagnetics 1996; 17:195-208. [DOI link]
Foster KR, Glaser R. Thermal mechanisms of interaction of radio frequency energy with biological systems with relevance to exposure guidelines. Health Phys 2007; 92:609-620. [DOI link]
Gabriel C, Gabriel S, Corthout E. The dielectric properties of biological tissues: I. Literature survey. Phys Med Biol 1996; 41:2231-2249. [DOI Link]
Gabriel S, Lau RW, Gabriel C. The dielectric properties of biological tissues: II. Measurements in the frequency range 10 Hz to 20 GHz. Phys Med Biol 1996; 41:2251-2269. [DOI Link]
Gabriel S, Lau RW, Gabriel C. The dielectric properties of biological tissues: III. Parametric models for the dielectric spectrum of tissues. Phys Med Biol 1996; 41:2271-2293. [DOI Link]
Hoult DI. Sensitivity and power deposition in a high-field imaging experiment. J Magn Reson Imaging 2000; 12:46-67. [DOI link]
Miklavčič, D, Pavšelj N, Hart FX. Electric Properties of Tissues. In: Wiley Encyclopedia of Biomedical Engineering, M. Akay (Ed), 2006:1-12. [DOI link]
Payne D, Klingenböck A, Kuster N. IT’IS Database for thermal and electromagnetic parameters of biological tissues, Version 4.0, 15 May 2018. [DOI Link]
Schwan HP, Li K. Capacity and conductivity of body tissues at ultrahigh frequencies. Proceedings of the IRE 1953; 41:1735–1740. [DOI link]
Related Questions
Is it safe to live under power lines, use a cell phone, or sleep under an electric blanket at night?
I've heard that "eddy currents" cause problems for imaging. What are they?
Does MRI pose any risk to the developing fetus? Do you scan pregnant patients?
Is it safe to live under power lines, use a cell phone, or sleep under an electric blanket at night?
I've heard that "eddy currents" cause problems for imaging. What are they?
Does MRI pose any risk to the developing fetus? Do you scan pregnant patients?